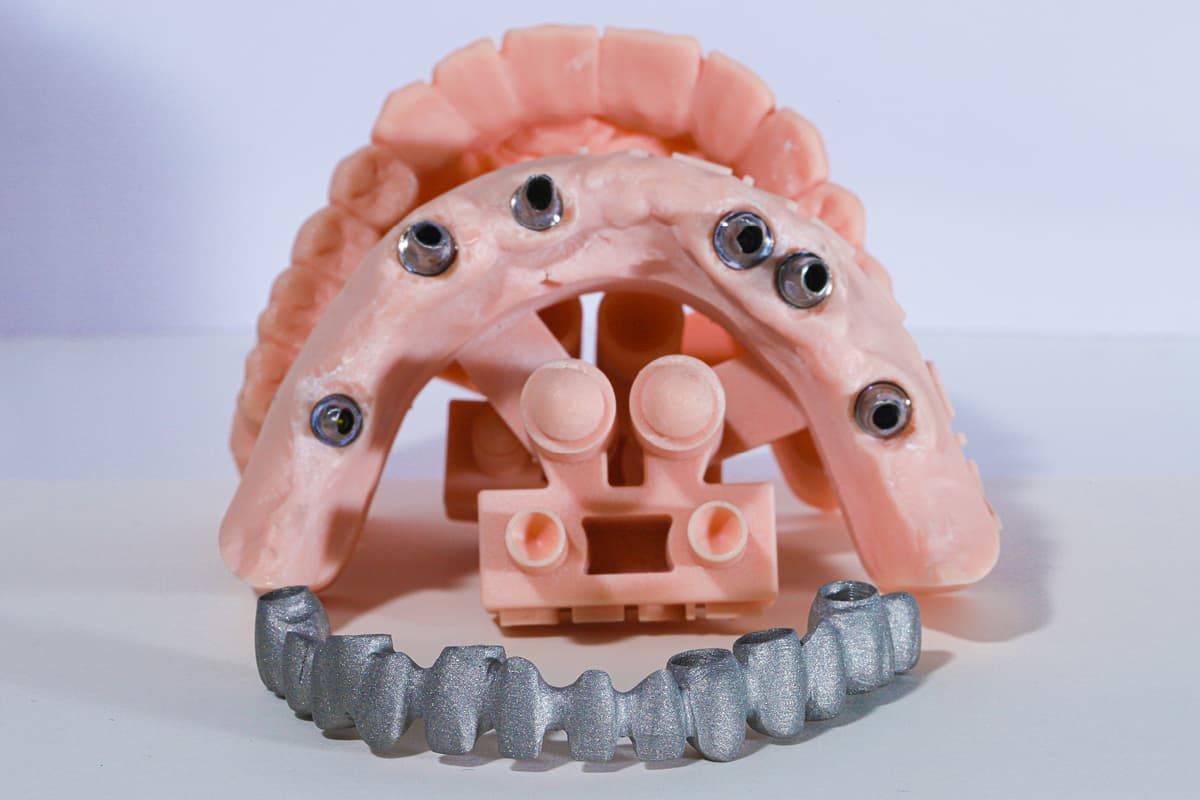
The creation of implant-supported prosthetic frameworks constitutes a fundamental technical phase for implant-prosthetic rehabilitation.(1,2) There are several techniques and materials that can be used for this purpose today.(3) The main methods of framework creation can essentially be broken down to two: traditional (analogue) techniques and digital techniques.(3)
In both cases, the aim is the same, that is, the creation of precise structures connecting the implant and the prosthesis or the prosthesis and the intermediate abutment (in the case of MUA, for example), in order to minimise the implant misfit, that is, any discrepancies in the fit of the connections that could cause biological and/or mechanical complications.(4,5)
Materials used for the construction of frameworks on implants
Various materials are used for the creation of frameworks on implants; among the most common, of course, are metal alloys such as chromium-cobalt (Co-Cr) and commercially pure titanium, valued for their mechanical and biological properties, as well as for the ease and multiplicity of methods that can be used to machine them.(6)
Alternative materials have also been introduced more recently, such as zirconia and polyetherketone (or PEEK), which are being used more and more successfully in implant-supported prostheses.(7)
Of course, the different types of materials are linked to different production techniques.
Metals, for example, can be processed with both analogue and digital techniques, while zirconia and PEEK can only be processed with digital techniques.(8)
Techniques for creating implant frameworks: analogue and digital
The traditional methods of manufacturing implant frameworks, of course, include lost wax casting.(9,10) This type of processing involves the creation of a wax model on a plaster model obtained from a conventional impression. Next, the wax that serves as a base for melting the metal is evaporated in a special oven, leaving space in the coating material for pouring the molten metal.
This process, used by the vast majority of dental technicians for years and still in use today, requires great attention both in the use of the various materials (plaster, coating materials, metal, etc.) and also in the design of the wax-up with the relative casting channels, where the molten metal is poured.
In this process, incorrect handling of materials and improper wax design can introduce errors in the precision with which the framework is produced. Furthermore, the thermal variation of the materials used (such as plasters, resins and metal alloys) exposed to very high temperatures still leads to a certain degree of imprecision.(9,10)
In this sense, the manual skill of the operator and the ability to prevent errors or minimise them, which in the prosthetic field are cumulative for the purposes of rehabilitation, are fundamental to maintaining constant precision in the production of implant-supported frameworks.(11)
CAD-CAM and 3D printing in the production of implant frameworks
Digital manufacturing methods, on the other hand, include CAD-CAM (Computer-Aided Design and Computer-Aided Manufacturing) methods, which are rapidly replacing analogue techniques thanks to the reduction of processing times and the simultaneous improvement of precision in the production of devices.(12) These methods are divided into two main categories: subtractive technologies and additive technologies.(13)
Subtractive manufacturing involves the milling of a block of pre-formed or pre-sintered material (such as zirconia), while additive manufacturing uses technologies such as 3D printing in its various forms, such as Selective Laser Melting for metals or Digital Light Processing (DLP) for resins. Objects are created layer by layer starting from metal powders or resin liquids which are subsequently polymerized by a laser beam with specific characteristics.(14)
Additive technologies have some advantages over subtractive technologies, such as the ability to produce devices with complex geometries which are difficult to obtain with milling machines, the saving of material, and the elimination of milling cutters to produce an artefact which could alter its surface properties, especially when worn.(14,15) However, 3D printing technologies are much more sensitive to environmental, instrumental and structural design factors (16).
Implant frameworks created with 3D printing generally have more porous structures and inferior mechanical properties compared to those obtainable with milling, especially when compared with technologies such as selective laser sintering for metals. For this purpose, selective laser melting is more suitable, even if the metal structures at the end of the process are extremely micro-rough and therefore must be machined again in the connection area.(17) However, from an accuracy point of view, selective laser melting seems to guarantee a result at least equal to milling, if not even better in connection precision.(18,19)
After the framework has been manufactured, aesthetic coating procedures with ceramic or resin materials may or may not be planned. These treatments can influence the final fit of the prosthesis and the precision of the implant-prosthetic connection since the high temperature firing cycles, required for example by ceramics, can induce deformations in the structure due to the thermal expansion between the ceramic and the underlying metal structure.(20)
Coatings with acrylic resin can also influence the adaptation of the structure and increase discrepancies at the implant-abutment connection level, due to the volumetric retraction that occurs during polymerisation of the resin itself.(21)
Which technique to choose for prosthetic frameworks
In conclusion, both analogue and digital technologies play a crucial role in the creation of implant structures, offering advantages and possible problems at the same time.
Traditional techniques, although they require greater manual skill and present possible margins of imprecision linked to the materials and thermal processes, are still widely used. However, the advancement of digital methods, such as CAD-CAM and 3D printing, is rapidly transforming the industry with increased precision and time optimisation.
The choice of the most appropriate technology depends on the clinical needs and the characteristics of the materials used, but the primary objective remains the minimisation of misfit and the preservation of the biological and mechanical properties of the materials.
References:
- Ortensi L, Ortensi M, Minghelli A, Grande F. Implant-Supported Prosthetic Therapy of an Edentulous Patient: Clinical and Technical Aspects. Prosthesis [Internet]. 2020 Jul 1 [cited 2020 Sep 20];2(3):140–52. Available from: https://www.mdpi.com/2673-1592/2/3/13
- Scrascia R, Fiorillo L, Gaita V, Secondo L, Nicita F, Cervino G. Implant-Supported Prosthesis for Edentulous Patient Rehabilitation. From Temporary Prosthesis to Definitive with a New Protocol: A Single Case Report. Prosthesis [Internet]. 2020 Feb 10 [cited 2021 Feb 5];2(1):10–24. Available from: https://www.mdpi.com/2673-1592/2/1/2
- Alshehri H, Alotaibi H, Alshareef N, Alsenani N, Aljuma’ah L, Alshhrani W, et al. Effect of Framework’s Manufacturing Technique on Screw’s Preload of Implant Supported Prosthesis. Niger J Clin Pract. 2022 Feb;25(2):185–91.
- Abdelrehim A, Etajuri EA, Sulaiman E, Sofian H, Salleh NM. Magnitude of misfit threshold in implant-supported restorations: A systematic review. J Prosthet Dent. 2022 Nov 7;S0022-3913(22)00613-8.
- Abduo J, Judge R. Implications of Implant Framework Misfit: An Animal Study on an Ovine Model. J Oral Implantol. 2021 Jun 1;47(3):183–9.
- Svanborg P, Eliasson A, Stenport V. Additively Manufactured Titanium and Cobalt-Chromium Implant Frameworks: Fit and Effect of Ceramic Veneering. Int J Oral Maxillofac Implants. 2018;33(3):590–6.
- Nuytens P, Grande F, D’haese R, Salameh Z, Lepidi L. Novel complete-arch pillar system (CAPS) to register implant position and maxillomandibular relationship in one single visit. J Dent. 2024 Feb 10;143:104885.
- Abduo J. Fit of CAD/CAM implant frameworks: a comprehensive review. J Oral Implantol. 2014 Dec;40(6):758–66.
- McCoy T. Lost wax casting technique for metal crown fabrication. J Vet Dent. 2014;31(2):126–32.
- Dahl BE, Dahl JE, Rønold HJ. Internal fit of three-unit fixed dental prostheses produced by computer-aided design/computer-aided manufacturing and the lost-wax metal casting technique assessed using the triple-scan protocol. European Journal of Oral Sciences [Internet]. 2018 [cited 2024 Oct 24];126(1):66–73. Available from: https://onlinelibrary.wiley.com/doi/abs/10.1111/eos.12394
- Auškalnis L, Akulauskas M, Jegelevičius D, Simonaitis T, Rutkūnas V. Error propagation from intraoral scanning to additive manufacturing of complete-arch dentate models: An in vitro study. Journal of Dentistry [Internet]. 2022 Jun 1 [cited 2022 Oct 5];121:104136. Available from: https://www.sciencedirect.com/science/article/pii/S0300571222001920
- Gomes ÉA, Tiossi R, Faria ACL, Rodrigues RCS, Ribeiro RF. Torque loss under mechanical cycling of long-span zirconia and titanium-cemented and screw-retained implant-supported CAD/CAM frameworks. Clin Oral Implants Res. 2014 Dec;25(12):1395–402.
- Alghazzawi TF. Advancements in CAD/CAM technology: Options for practical implementation. Journal of Prosthodontic Research [Internet]. 2016 Apr [cited 2023 Mar 18];60(2):72–84. Available from: https://linkinghub.elsevier.com/retrieve/pii/S1883195816000098
- Dawood A, Marti Marti B, Sauret-Jackson V, Darwood A. 3D printing in dentistry. Br Dent J. 2015 Dec;219(11):521–9.
- Jin G, Shin SH, Shim JS, Lee KW, Kim JE. Accuracy of 3D printed models and implant-analog positions according to the implant-analog–holder offset, inner structure, and printing layer thickness: an in-vitro study. Journal of Dentistry [Internet]. 2022 Oct 1 [cited 2024 Jul 25];125:104268. Available from: https://www.sciencedirect.com/science/article/pii/S0300571222003244
- Piedra-Cascón W, Krishnamurthy VR, Att W, Revilla-León M. 3D printing parameters, supporting structures, slicing, and post-processing procedures of vat-polymerization additive manufacturing technologies: A narrative review. J Dent. 2021 Jun;109:103630.
- Zhou Y, Wei W, Yan J, Liu W, Li N, Li H, et al. Microstructures and metal-ceramic bond properties of Co-Cr biomedical alloys fabricated by selective laser melting and casting. Materials Science and Engineering: A [Internet]. 2019 Jun [cited 2023 Sep 27];759:594–602. Available from: https://linkinghub.elsevier.com/retrieve/pii/S0921509319307178
- Revilla-León M, Ceballos L, Martínez-Klemm I, Özcan M. Discrepancy of complete-arch titanium frameworks manufactured using selective laser melting and electron beam melting additive manufacturing technologies. The Journal of Prosthetic Dentistry [Internet]. 2018 Dec 1 [cited 2023 Feb 6];120(6):942–7. Available from: https://www.sciencedirect.com/science/article/pii/S0022391318301847
- Revilla-León M, Ceballos L, Özcan M. Implant Prosthodontic Discrepancy of Complete-Arch Co-Cr Implant Frameworks Manufactured Through Selective Laser Melting Additive Manufacturing Technology Using a Coordinate Measuring Machine. Int J Oral Maxillofac Implants. 2019;34(3):698–707.
- Revilla-León M, Sánchez-Rubio JL, Pérez-López J, Rubenstein J, Özcan M. Discrepancy at the implant abutment-prosthesis interface of complete-arch cobalt-chromium implant frameworks fabricated by additive and subtractive technologies before and after ceramic veneering. J Prosthet Dent. 2021 May;125(5):795–803.
- Revilla-León M, Pérez-López J, Barmak AB, Raigrodski AJ, Rubenstein J, Galluci GO. Implant-Abutment Discrepancy Before and After Acrylic Resin Veneering of Complete-Arch Titanium Frameworks Manufactured Using Milling and Electron Beam Melting Technologies. J Prosthodont. 2022 Mar;31(S1):88–96.
Would you like more information about Zhermack Dental products and solutions?
Contact us